There are quite a few ways in which the human brain suggests to us that scientists are dead wrong when they claim that the brain is the source of our minds and dead wrong when they claim the brain is the storage place of our memories. Below is a discussion of the eleven biggest shortfalls of the brain suggesting that such claims are very much in error.
Neural Shortfall #1: The Lack of Any Stable Place in a Brain Where Learned Information Could Be Stored for Decades
Humans can hold memories for 50 years or longer. If you are to explain such memories under the idea that brains store memories, there needs to be a place in the brain that can store memories for 50 years or longer. There is no such place in the brain.
Here we have a gigantic failure of the most common idea of neural memory storage, the groundless claim that memories are stored in synapses. The proteins in synapses are very short-lived, having lifetimes of two weeks or shorter. Synapses do not last for years. Synapses are so small that it is all but impossible to track how long they last. But we know that synapses are connected to little bumps on dendrites, called dendritic spines, which are much larger than synapses. Scientists can track how dendritic spines change over time. Such observations have shown that dendritic spines are short-lived, usually lasting no more than a few weeks or months, and seemingly never lasting for years.
With the exception of the DNA inside the nucleus of neurons, neurons offer no place for stable information storage. In theory, you could store information for decades in the DNA in the nucleus of a neuron. But there is no sign that human learned information is ever written to DNA. When doctors extract some part of a brain to reduce seizures, they find neurons that all have the same information in their DNA: mere genetic information that is basically the same in every cell, including cells from your feet. The genetic code used by DNA is merely a code allowing for the representation of amino acids, not a code for allowing the representation of the things human learn in school.
Neural Shortfall #2: The Lack of Any Mechanism in the Brain for Writing Learned Information
We know how a computer stores information. There is a spinning hard disk, and what is called a read/write head. The head is a tiny unit capable of writing to any point on the disk, and reading from any point on the disk. Both the disk and the read/write head can move, which allow the read/write head to be positioned over any point on the disk, so that data can be read from anywhere on the disk, or written to anywhere on the disk.
If brains store memories, a brain would need to have some kind of mechanism for writing a new memory. But no such mechanism seems to exist. There is no kind of "read unit" or "write unit" that moves around from place to place on a brain to read and write.
In this regard neuroscientists are empty-handed. Don't be fooled by claims that the artificial effect called long-term potentiation is some kind of mechanism for memory. What is misleadingly called “long-term potentiation” or LTP is a not-very-long-lasting effect by which certain types of high-frequency stimulation (such as stimulation by electrodes) produces an increase in synaptic strength. Synapses are gaps between nerve cells, gaps which neurotransmitters can jump over. The evidence that LTP even occurs when people remember things is not very strong, and in 1999 a scientist stated (after decades of research on LTP) the following:
"[Scientists] have never been able to see it and actually correlate it with learning and memory. In other words, they've never been able to train an animal, look inside the brain, and see evidence that LTP occurred."
Since it does not last for years, and is almost always a very short-term effect, LTP cannot be some mechanism by which permanent memories are written to the brain. A recent scientific paper states this:
"It actually remains to be demonstrated that LTP = memory in most mammalian learning models. In fact, most studies of long-term plasticity do not explore beyond an hour or two; clearly not enough to establish a direct link with long-term memory formation."
Neural Shortfall #3: The Lack of Any Mechanism in the Brain for Reading Learned Information
There is in the brain no sign of any read mechanism. We can imagine what a read mechanism might look like in a brain if a brain stored memories. It might be some little unit that moved around from place to place in the brain. When such a read unit came to some place in the brain where data was to be read, the read unit might linger for a while until the data was read. Nothing like any such unit exists in the brain. The only thing that moves around in the brain is blood and chemicals that pass between synapses. There is no sign of anything like a read unit that moves around from one place to another in the brain to read information. There is no sign of anything like a scan mechanism in the brain.
Neural Shortfall #4: The Lack of Any Signal Transmission Mechanism in the Brain Fast Enough to Account for Instant Human Recall and Fast Thinking
In a post entitled "Authorities Spur Wrong Ideas About the Complexity of Proteins" on one of my other blogs, I discussed how search engines such as Google give you misleading ideas when you perform a simple search trying to find out how complex are the protein molecules inside our body. Something very similar happens when you search for how fast brain signals travel. If you type in a Google search phrase of "speed of brain signals" you get as the first line a sentence saying "The brain can send signals to the body at up to 100 meters per second." But you will commit a huge error if you remember that speed as "the speed of brain signals."
Such a speed is the fastest speed that signals can ever travel in a brain, when traveling across myelinated axons. But it is not at all the average speed of signal transmission in the brain. Almost all signal transmission in the brain occurs through a very much slower process involving transmission through chemical synapses (by far the most common type of synapses in the brain). Transmission across chemical synapses is relatively slow. Each time a signal crosses a chemical synapse, there is a delay called a synaptic delay. This is a delay between about .5 millisecond to 1 millisecond. The problem is that the brain is filled with as many as 100 trillion synapses, with as many as a thousand synapses for each neuron. To move through a few inches of brain tissue, a signal would have to cross very many synapses, resulting in a very substantial delay. In my post here I explain why the cumulative delay of having to travel across many synapses means that the average speed of brain signals should be no greater than about 1 centimeter per second (about .4 inches per second), which is about the speed of a snail.
A 2015 paper describes the speed of the transmission of various brain signals, without considering synaptic slowing factors. It lists these brain signal speeds:
"Interestingly, signal propagation speeds in various conditions are similar (~0.1 m/s). Neural spikes generated by 4-aminopyridine (4-AP) travel with a longi-tudinal speed of 0.09/0.03 m/s along the CA3 region (Kiblerand Durand, 2011), whereas in the presence of picrotoxin, synchronous firing events propagate longitudinally at 0.14 /0.04m/s (Miles et al., 1988). High K+-, low Mg2+-, and zero-Ca2+- triggered spikes again exhibit speeds of 0.07-0.1 m/s, 0.1– 0.15m/s, and 0.04 – 0.15 m/s, respectively (Haas and Jefferys, 1984;Quilichini et al., 2002;Liu et al., 2013). In normal tissue, theta oscillations travel with a speed of 0.08 – 0.107 m/s in the hip-pocampus of living rodent rats (Lubenov and Siapas, 2009),whereas carbachol-induced theta oscillations travels with a speed of 0.119 m/s along the CA1 cell layer and a 0.141 m/s along the CA3 cell layer (Cappaert et al., 2009). Together, it is clear that 0.1m/s is a common propagation speed regardless of experimental models...Other propagation mechanisms, such as extracellular ionic transients and axonal conduction mechanisms, have very different propagation speeds (0.0004 – 0.008 m/s for K+ diffusion and 0.3– 0.5 m/s for axonal conduction, Miles et al., 1988;Lian et al., 2001;Francis et al., 2003;Meeks and Mennerick, 2007;Jensen, 2008;Kibler et al., 2012)."
Given such numbers, my estimate above for an average brain signal speed transmission of only about 1 centimeter per second seems roughly correct. Some of the speeds quoted above are about five to ten times faster than my estimate, but the estimate for K+ diffusion (potassium diffusion) is much slower than my estimate. Most of the numbers quoted above are about 1000 times slower than the incorrect "100 meters per second" figure often given for brain signal speed. The speeds quoted above are "fastest time" quotes that don't consider all the slowing produced by cumulative synaptic delays and other slowing factors.
The 2021 paper "Brain Activity Fluctuations Propagate as Waves Traversing the Cortical Hierarchy" mentions an average speed of about 10 millimeters per second, which is very close to the rough estimate I give above of a brain signal speed of about 1 centimeter per second (10 millimeters per second is the same as 1 centimeter per second). We read this: "The top-down propagations (N = 8519) and bottom-up propagations (N = 18 114) were found to account for 9.08% and 19.7% of the total scanning time, respectively, with an average speed of 13.45 ± 7.78 and 13.74 ± 7.51 mm/s (mean ± SD), respectively." We should very carefully note that the average speed of signals in the brain is way too slow to account for blazing-fast human thinking and instant human recall. See my post here for examples of exceptionally fast thinkers who calculated so fast we could never explain their results by imagining brain signals traveling at sluggish speeds such as 1 centimeter per second.
Neural Shortfall #5: The Lack of Any Encoding System by Which Brains Could Translate Learned Information Into Synapse States or Neural States
Just as there is pretty much no such thing as “just storing” information on a computer, without using some type of encoding, there could be no such thing as “just storing” a memory in the brain. For a brain to store memories, it would have to do some encoding by which sensory information or thought information was converted into neural states or synapse states. Neuroscientists love to keep using the word "encoding" when referring to memory, such as saying that memory formation begins with encoding. But neuroscientist have not the slightest understanding of how a brain could encode the type of things that humans experience and learn.
When words are stored on a computer or smartphone, multiple levels of encoding are involved. First, there is the alphabetic encoding. Then there is what is called an ASCII encoding. The individual letters are converted to numbers, using an ASCII table that signifies how particular letters will be stored as particular numbers (an example of an ASCII table is below). Then there is a binary encoding by which numbers such as 34 written in the base 10 system are converted to a series of 1's and 0's such as 100101101.
ASCII is an encoding protocol
We see in the diagram below three different types of encoding going on when the word “cat” is stored on a computer.
When an image is stored on a computer, there are also multiple types of encoding going on. The image is broken down into a grid of pixels; each pixel is translated into a number indicating a particular shade of color; then those numbers are translated into binary form that is ideal for storing on a computer hard drive.
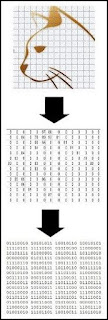
Encoding of a visual image
Now let us consider how the brain might store memories. If we are to imagine that the brain stores memories, we must imagine that the brain somehow does encoding to get the memory stored to the brain. But for a brain to store everything humans learn and experience, it would seem to need not just one encoding protocol, but many different encoding protocols. This is because of the vast variety of things humans can learn and experience, which range from auditory to visual to purely conceptual, and which range from experiential (involving self-experience) to accounts of other people's experience. One very big problem is that if this magnificent suite of encoding protocols existed, it would be a "miracle of design" that would vastly worsen the problem of explaining a natural origin of humans.
But the biggest problem is that there simply is no sign of any such protocols being used anywhere in the brain. Scientists are unable to discover the slightest trace of any such protocols in the brain. Could it be that microscopic encoding needed to encode memories in brain would just be too hard to discover? No, that idea does not hold water. Scientists were able in the time around 1948 to 1960 to discover a microscopic encoding protocol used in the human body: the genetic code, by which triple combinations of nucleotide base pairs stand for amino acids. If the brain was doing multiple types of encoding by which human experiences and human learned knowledge were converted into brain states or synapse states, that would have left a "footprint" that scientists would have been able to discover half a century ago. Now the microscope technology of scientists is vastly greater than the technology that was able to discover the genetic code about 1950. But still neuroscientists have found no signs of any code by which human experiences and human learned knowledge could be translated into neural states.
Neural Shortfall #6: The Lack of Any Region of the Brain With Activity Strongly Correlating With Thinking, Memory Formation or Recall
A recent story in the Sunday Times of London is a showcase for a variety of objectionable claims and behavior by today's neuroscientists. The story (which I won't link to because it's behind a paywall) is entitled "The brain scientist who wants to zap away your depression." We have again the appalling practice of subjecting a journalist to some medically unnecessary brain treatment, presumably so that the journalist can act like some "embedded journalist" who writes favorable stories about those he has been entangled with. Here is a sickening quote about some brain zapping that is not part of a funded scientific experiment and has no medical value for the subject:
" 'This might a little bit uncomfortable,' she says, pressing the button. Tap, tap, tap, tap. Repeated jolts fire into my brain. Pause. Tap, tap, tap, tap. My face screws up as the muscles in my cheek spasm. It does not hurt but it certainly is not pleasant."
We have a long plug for a book written by a neuroscientist telling us the nonsensical idea that everything is measurable. The neuroscientist makes the ludicrous claim, "I don't think depression is a real natural phenomenon." She also makes the very misleading insinuation that we've got mental health wrong because people aren't emphasizing brains, a claim contrary to the truth that chemical and neuroscience approaches have been the main approach taken for decades, with neuroscience failing to deliver for psychiatry. As part of the article we have one of those "function localization" charts claiming that particular parts of the brain have particular cognitive functions.
There is no robust evidence for such claims. The
lack of any region of the brain with activity strongly correlating
with thinking, memory formation or recall is one of many reasons for rejecting the claim that the brain is the source of thinking and the storage place of memories. For posts discussing this lack, see my post "The Brain Shows No Sign of Working Harder During Thinking or Recall," my post "Studies Debunk Hippocampus Memory Myths," and my post "Reasons for Doubting Thought Comes from the Frontal Lobes or Prefrontal Cortex." For a discussion of the "lying with colors" visuals that neuroscientists repeatedly use to create misleading impressions of much greater activity in brain regions without significantly greater activity during particular activities, see my post "Neuroscientists Keep Using Misleading Coloring in Brain Visuals."
Neural Shortfall #7: Very High Brain Signal Noise, and the Lack of Any Capability in the Brain for Reliably Transmitting Signals Throughout Brain Tissue
A 2020 paper states this:
"Neurons communicate primarily through chemical synapses, and that communication is critical for proper brain function. However, chemical synaptic transmission appears unreliable: for most synapses, when an action potential arrives at an axon terminal, about half the time, no neurotransmitter is released and so no communication happens... Furthermore, when neurotransmitter is released at an individual synaptic release site, the size of the local postsynaptic membrane conductance change is also variable. Given the importance of synapses, the energetic cost of generating action potentials, and the evolutionary timescales over which the brain has been optimized, the high level of synaptic noise seems surprising."
Such a result (a very serious brain physical shortfall) is surprising only to those who believe that your brain stores your memories and that your brain makes your mind. Those who disbelieve such a thing may expect exactly such shortfalls to be repeatedly found.
The quote above states one of the most important shortfalls of the human brain: that chemical synapses (by far the most common type) do not reliably transmit signals, and transmit signals with an accuracy of 50% or less. This is a fact with the most gigantic consequences. Given that brain signals have to travel across many different synapses, it should be impossible for humans to recite any long bodies of textual information with even 50% accuracy. But instead of having such dismal recall abilities, humans again and again show the ability to perfectly recite very large bodies of memorized information. This occurs every time an actor recalls all of the 1422 lines in the role of Hamlet, and every time a Wagnerian opera singer correctly sings all of the words and notes of the very long roles of Siegfried or Hans Sachs. It occurs even more dramatically when certain Moslem memory marvels correctly recall all of the 6000+ lines in their holy book. For many similar cases, see my post "Exceptional Memories Strengthen the Case Against Neural Memory Storage."
Below is an example of the type of result in a high-noise system that does not reliably transmit information.
Neural Shortfall #8: The Lack of Any Token Repetition in the Brain Other Than the Nucleotide Base Pair Tokens Capable of Representing Only Low-Level Chemical Information Such as Sequences of Amino Acids
Even when there is an encoding system that has not yet been deciphered or figured out, there will be indications that such an encoding system exists. The indications are repeated tokens. Before Europeans were able to figure out how hieroglyphics worked, they knew that hieroglyphics used some type of encoding system, because they could see how frequently there was a repetition of individual tokens or symbols. Not counting the genetic information stored in neurons and many other types of cells, where we do see an enormous amount of token repetition, there is no sign of any token repetition in the brain. This is a clear sign that the brain does not use any system of encoding to store human memories. And if there is no such system of encoding, we should not think that brains store memories; for some system of encoding would be required to convert human experiences and human learned knowledge into brain states.
Neural Shortfall #9: The Lack of Any Discovered Stored Memories in Extracted Brain Tissue or Dissected Corpses
Within cells below the neck, there is stored information in DNA using a system of representations called the genetic code. Scientists were able to discover such a system in the middle of the 20th century, and were able to start recovering information stored using such a system, which was all low-level chemical information such as which amino acids make up particular proteins. If the human brain stored memories, there would be brain areas storing memories that could be studied microscopically to find what a person had learned. You would then be able to find out some of the things someone had learned or experienced by studying his brain. No such memories have ever been discovered by microscopically examining brain tissue, even though scientists today have vastly been microscopes than they had in the 1950's.
Neural Shortfall #10: The Lack of Any Mechanism Allowing a Very Rapid Transformation of Brain Tissue or Synapses Sufficient to Account for Instant Memory Formation
It is a fact of human experience that humans can form permanent new memories instantly. A successful theory of a brain storage of memories would somehow have to account for this fact, by explaining how permanent traces of an experience could instantly appear. There is no theory of a brain storage of memory that does such a thing. Theories of a brain storage of memory (which are little more than hand-waving and catchphrases) typically appeal to protein synthesis as a key part of memory formation. But protein synthesis requires quite a few minutes. The shortfall of neuroscientists in this regard is so great that they typically end up making ridiculous claims (contrary to all human experience) that it takes minutes for a person to form a new memory.
Neural Shortfall #11: The Lack of Any Indexing System or Coordinate System or Addressing System That Could Help to Explain Instant Memory Recall
It is a fact of human experience that humans recall correct answers instantly. If you ask me what was the biggest conquest of Julius Caesar, I don't have to take minutes or hours waiting for my brain to search for the answer. Instead, the instant you finish asking the question, I will give the correct answer: the Gauls in France. How can humans answer questions so quickly? We know that indexing can make possible very fast retrieval of information such as occurs in database systems. But the brain has no sign of having any indexing system. An indexing system can only occur if there exists an addressing system or a position notation system (for example, a book has the position notation system called page numbering, and the index at the back of the book leverages that position notation system). Brains have neither an indexing system nor an addressing system nor the position notation system that is a prerequisite for an indexing system. There are no neuron numbers and no neural coordinate system that a brain might use to allow fast, indexed retrieval of a memory. So there's no way to explain how instant recall could occur if you are retrieving memories from your brain.
We don't think and recall at the sluggish speed of brains; we think and recall at the speed of souls.
BRAIN FACT | IMPLICATION, RELATED FACT OR OBSERVED CONSEQUENCE | SHORTFALL OF ALL BRAINS | IMPLICATION |
Neurons, synapses and dendrites are physically rooted in fixed spots in the brain, like trees rooted in one spot in a forest | There can be no physical sorting in the brain |
|
|
Neurons have no addresses and no neuron numbers | There can be no indexing in the brain, as indexes require addresses or position numbers | Brains have no sorting, indexing or addresses | Brains cannot explain instant detailed recall, when someone instantly recalls lots of relevant information when hearing a name or seeing a face |
Synapses and dendritic spines are made of proteins with short lifetimes (< 2 weeks) | Synapses are attached to dendritic spines, observed to be unstable, lasting for less than years | Synapses are not a stable medium for storing human memories that can last reliably for 50+ years | The synaptic theory of brain memory storage is untenable |
There are no moving anatomical parts in the brain | There are no moving anatomical parts in the brain corresponding to learning | Brains have no mechanism for writing learned information | Learning of conceptual knowledge cannot be explained by brain mechanisms |
Transmission of signals between neurons requires passing through endless chemical synapses (~1000 chemical synapses for every neuron) | Chemical synapses do not reliably transmit brain signals (action potentials), transmitting them successfully only 10% to 50% of the time. Brains have no structure supporting the storage of large learned sequences. | Large amounts of precise sequential information (such as large bodies of text) cannot be reliably transmitted across brain regions | A feat which many humans can perform (the exact retrieval of large bodies of text) cannot be achieved by a retrieval of stored information in a brain. |